Electron Transport Chain: Understanding the Sequence in Aerobic Respiration
The electron transport chain in aerobic respiration
Aerobic respiration is the primary process by which organisms convert nutrients into energy. At the heart of this process lie the electron transport chain (etc.), where electrons travel downward sloping through a specific sequence of carriers. This energetically favorable movement drive ATP synthesis, provide cells with the energy currency need for near all biological functions.
The electron sequence: an overview
During aerobic respiration, electrons travel downward sloping (from high to low energy states )in a specific sequence through protein complexes embed in the inner mitochondrial membrane. This sequence is:

Source: chegg.com
- NADH / fadeâ‚‚ (electron donors )
-
Complex i (nNADHdehydrogenase )or complex ii ( (ccinate dehydrogenase )
) - Ubiquitous (coenzyme q )
- Complex iii (cytochrome bcâ‚ complex )
- Cytochrome c
- Complex iv (cytochrome c oxidase )
- Oxygen (final electron acceptor )
This pathway represents a cautiously orchestrate energy transfer system where electrons move from carriers with more negative reduction potentials to those with more positive reduction potentials.
Electron donors: where the journey begin
The electron transport chain begin with high energy electron carriers NADH and fade₂, which are produce during earlier stages of cellular respiration – glycolysis, pyruvate oxidation, and the citric acid cycle.
NADH delivers its electrons to complex i( NADH dehydrogenase), while ffade deliver electrons to complex ii ((uccinate dehydrogenase ))This reprepresentse first step in the downhill electron journey, as these molecules have the nigh negative reduction potentials in the chain.
The energy difference between NADH and oxygen is substantial – roughly 320 mMVfor nNADHcompare to +820 mMVfor oxygen. This significant energy gradient drive the entire process.
Complex i: NADH dehydrogenase
Complex I is the first and largest protein complex in the electron transport chain. When NADH bind to complex i, it transfers two electrons and isoxidizede binding tNADadâº. The complex contain a flavin mononucleotid((FMN)) that initially accept these electrons.
As electrons move through complex I’s iron sulfur clusters, their energy drives the pumping of four hydrogen ions( protons) from the mitochondrial matrix into the intermembrane space. This proton will pump will contribute to the electrochemical gradient that will finally will power aATPsynthesis.
The electrons finally transfer to ubiquitous (coenzyme q ) which carry them to the next complex.
Complex ii: succinate dehydrogenase
Complex ii represent an alternative entry point for electrons into the transport chain. This complex oxidizes succinate to fumarate as part of the citric acid cycle, generatefadehâ‚‚ in the process.
Fadeâ‚‚ so transfer its electrons to iron sulfur clusters within complex ii, which after pass them to ubiquitous. Unlike complex i, complex ii does not pump protons across the membrane because the energy difference between fadeâ‚‚ and ubiquitous is insufficient to drive this process.
This explains whyfadeh₂ generate lessATPp thanNADHh – electrons fromfadeh₂ enter the chain at a lower energy level, bypass one proton pump site.
Ubiquitous: the mobile electron carrier
Ubiquitous (coenzyme q or cCOQ)is a small, lipid soluble molecule that freely diffuse within the inner mitochondrial membrane. It seservess a mobile electron carrier, collect electrons from both complex i and complex ii.
When ubiquitous accept electrons, it’s reduce to ubiquitous( ohâ‚‚). This reduced form so will diffuse through the membrane to complex iii, where it’ll donate its electrons to will continue the transport chain.
The mobility of ubiquitous is crucial for connecting the various protein complexes embed in the membrane, allow for efficient electron flow between them.
Complex iii: cytochrome bcâ‚ complex
At complex iii, ubiquitous donate its electrons through a process call the q cycle. This complexcontainsn multiple cytochrome proteins with heme groups that facilitate electron transfer.
The q cycle is a sophisticated mechanism that allow complex iii to pump four protons into the intermembrane space for every pair of electrons that pass through. This contributes importantly to the proton gradient across the membrane.
As electrons will move through complex iii, they’re finally will transfer to will cytochrome c, another mobile carrier that will shuttle them to the final complex in the chain.
Cytochrome c: the second mobile carrier
Cytochrome c is a small, water-soluble protein locate in the intermembrane space. It contains a heme group that can accept and donate a single electron.
After receive electrons from complex iii, cytochrome c diffuse along the outer surface of the inner mitochondrial membrane until it reach complex iv. Thither, it donates its electrons to continue their downhill journey.
Unlike ubiquitous, which can carry two electrons, cytochrome c transfers electrons one at a time, require two cytochrome c molecules to transfer the pair of electrons from each ubiquitous.
Complex iv: cytochrome c oxidase
Complex iv represent the final protein complex in the electron transport chain. It receives electrons from cytochrome c and transfer them to their ultimate destination: molecular oxygen( oâ‚‚).
This complex contains copper centers and additional cytochromes that facilitate the four electron reduction of oâ‚‚ to form two water molecules:
O₂ + 4e⻠+ 4h⺠→ 2h₂o
As electrons move through complex iv, their energy drives the pumping of two protons across the membrane,airr contribute to the electrochemical gradient.
Oxygen: the final electron acceptor
Oxygen serve as the final electron acceptor in aerobic respiration, give the process its name. With its strong electronegativity and high reduction potential (+820 mMV) oxygen pull electrons through the entire transport chain.

Source: numerade.com
The reduction of oxygen to water is extremely exergonic, release substantial energy that drive the proton pumping activities of the electron transport complexes. This make oxygen an ideal terminal electron acceptor for maximize energy extraction.
Without oxygen, the electron transport chain would rapidly back up as electrons accumulate in the carriers, halt the process. This explains why we need to breathe ceaselessly – to supply our cells with the oxygen necessary to maintain electron flow.
The proton gradient and ATP synthesis
As electrons move downward sloping through the transport chain, their energy drives the pumping of protons from the mitochondrial matrix into the intermembrane space. Thiscreatese both a concentration gradient and an electrical gradien(( unitedly call the electrochemical gradient or proton motive forc)).
This gradient represents store energy, similar to water behind a dam.ATPp synthase, oftentimes call complex v, harness this energy as protons flow backwards into the matrix through its channel. This flow drive the rotation of parts of the enzyme, catalyze the synthesis ofATPp fromADPp and inorganic phosphate.
For each pair of electrons that travel from NADH to oxygen, enough protons are pump to generate around 2.5 ATP molecules. Electrons from fadeâ‚‚ yield around 1.5 ATP molecules, reflect their entry at a lower energy level in the transport chain.
Redox potentials: the energy gradient
The electron transport chain functions because of differences in reduction potentials between the carriers. Each component have a characteristic standard reduction potential (e ° ′ )measure in volts or millivolts.
The sequence of electron carriers is arranged in order of progressively positive reduction potentials:
- NADH / NADâº: 320 mMV
- Fadeâ‚‚/fad: 220 mMV
- Ubiquitous: +45 MV
- Cytochrome c: +235 MV
- Oxygen / water: +820 MV
This arrangement ensure that electrons move impromptu from carriers with more negative potentials to those with more positive potentials, release energy at each step.
Inhibitors of the electron transport chain
Various compounds can disrupt the electron transport chain by bind to specific complexes and block electron flow. Understand these inhibitors has been crucial for elucidate the sequence of electron carriers:
-
Rotenone and Amytal
Inhibit complex i -
Malone
Inhibit complex ii -
Antimycin a
Block electron transfer in complex iii -
Cyanide, aside, and carbon monoxide
Inhibit complex iv by bind to the oxygen reduction site -
Oligomycin
Block ATP synthase, prevent proton flow through the enzyme
These inhibitors are much lethal because they prevent ATP production, highlight the essential nature of the electron transport chain for cellular energy generation.
Evolutionary significance of the electron transport chain
The electron transport chain represents one of thewell-nighh ancient and conserved metabolic pathways. Its development potential coincide with the rise of atmospheric oxygen around 2.5 billion yearsalonee, revolutionize energy production in early organisms.
Before oxygen was available as an electron acceptor, primitive organisms rely on fermentation and anaerobic respiration, which yield importantly less energy. The ability to use oxygen provide a tremendous selective advantage, allow for the evolution of more complex multicellular organisms with higher energy demands.
Mitochondria themselves originate from free live bacteria that were engulfed by larger cells in an endosymbiotic relationship. Thiexplainsin why the electron transport chain locatedate in the mitochondrial membrane instead than elsewhere in the cell.
Clinical relevance of the electron transport chain
Dysfunction in the electron transport chain is implicated in numerous human diseases and conditions:
-
Mitochondrial diseases
Much result from mutations in genes encode components of the electron transport chain -
Neurodegenerative disorders
Like Parkinson’s and Alzheimer’s show evidence of mitochondrial dysfunction -
Age
Is associate with decline efficiency of the electron transport chain -
Ischemia reperfusion injury
Occur when oxygen supply is restored after a period of oxygen deprivation, lead to excessive reactive oxygen species production
Understand the precise sequence and mechanism of electron flow has been crucial for developing therapeutic approaches to these conditions.
Summary: the complete electron journey
To summarize the downhill sequence of electron travel during aerobic respiration:
- High energy electrons from nutrient breakdown are capture by NAD⺠and fad to form NADH and fade₂
- NADH donate electrons to complex i, while fadeâ‚‚ donate to complex ii
- Both complexes transfer electrons to ubiquitous (coenzyme q )
- Reduced ubiquitous (uubiquitou) carry electrons to complex iii
- Complex iii transfers electrons to cytochrome c
- Cytochrome c shuttle electrons to complex iv
- Complex iv use the electrons to reduce oxygen to water
Throughout this journey, the energy release from the electrons flow downward sloping drive proton pumping across the inner mitochondrial membrane, create an electrochemical gradient that powers ATP synthesis via ATP synthase.
This elegant system demonstrate how cells harness the fundamental principles of thermodynamics and electrochemistry to convert the chemical energy in food into the usable energy that power life.
MORE FROM searchhole.com
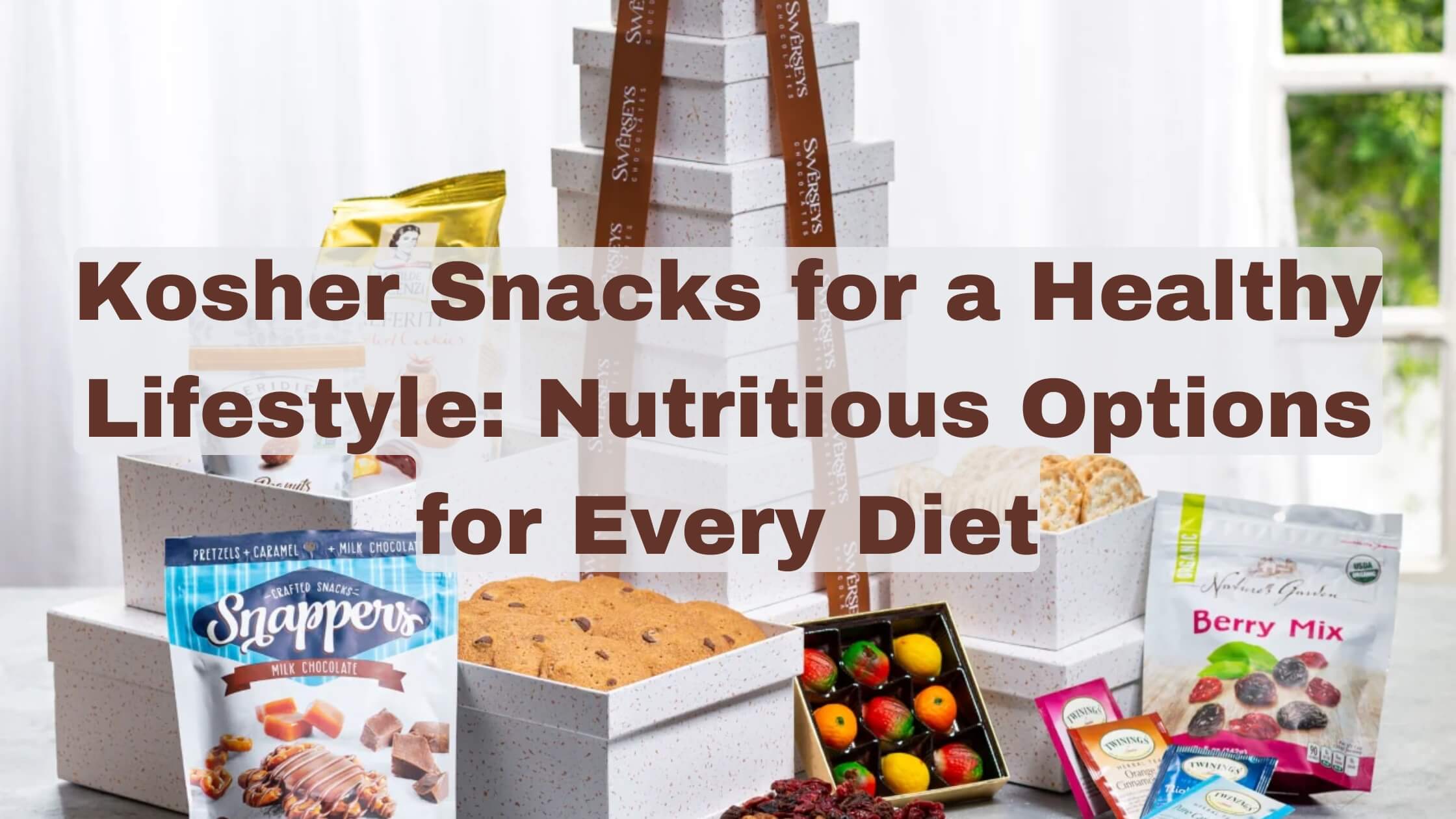
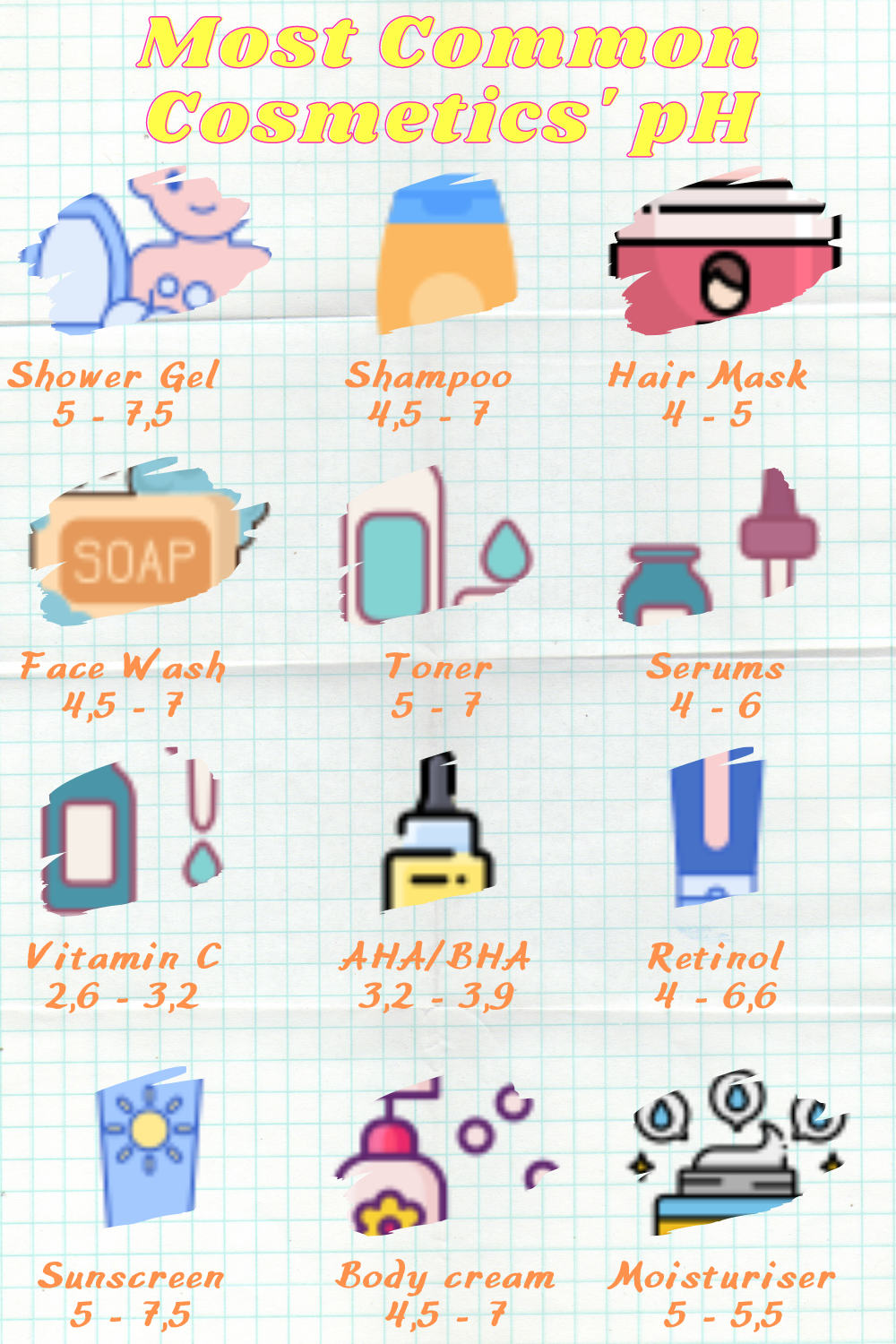
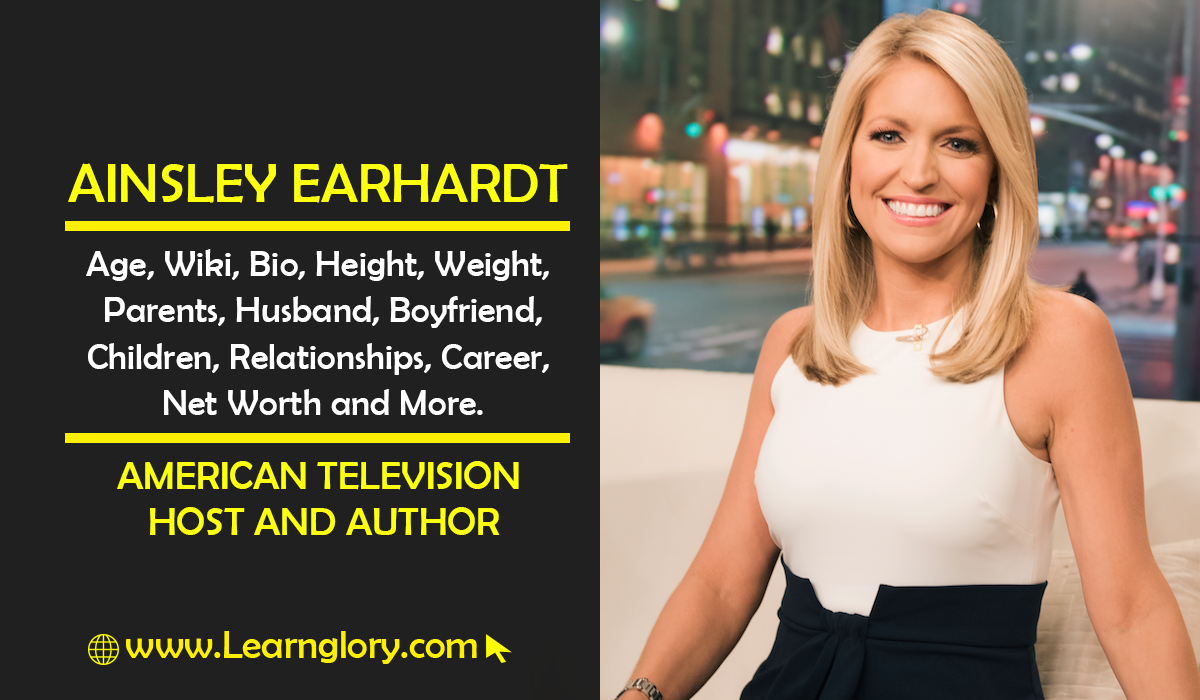
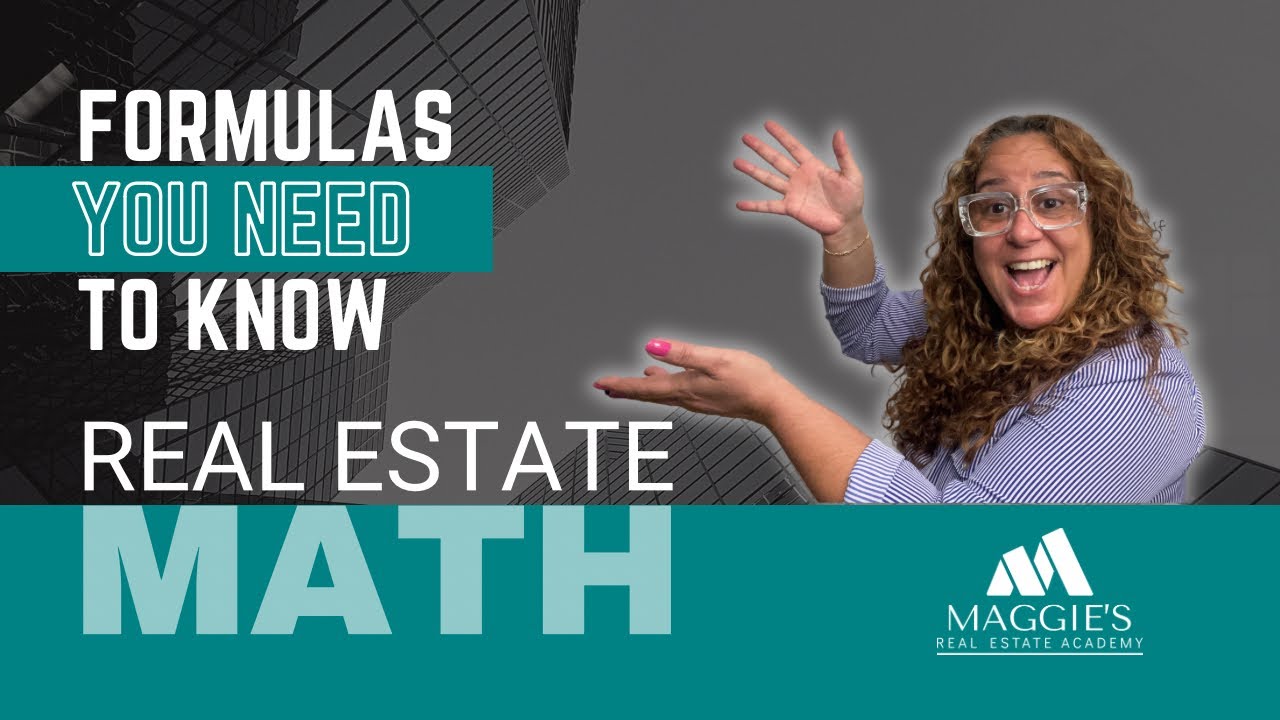
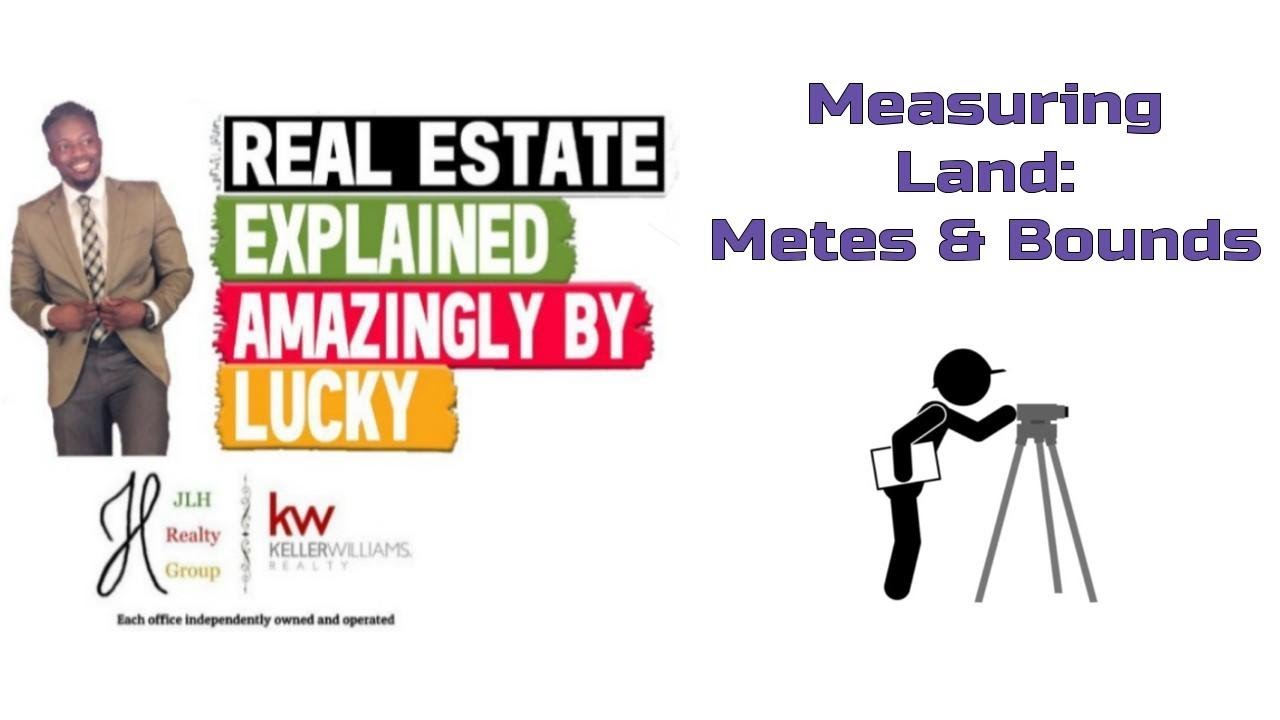
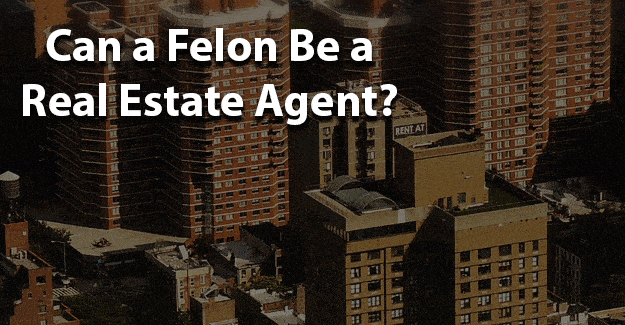
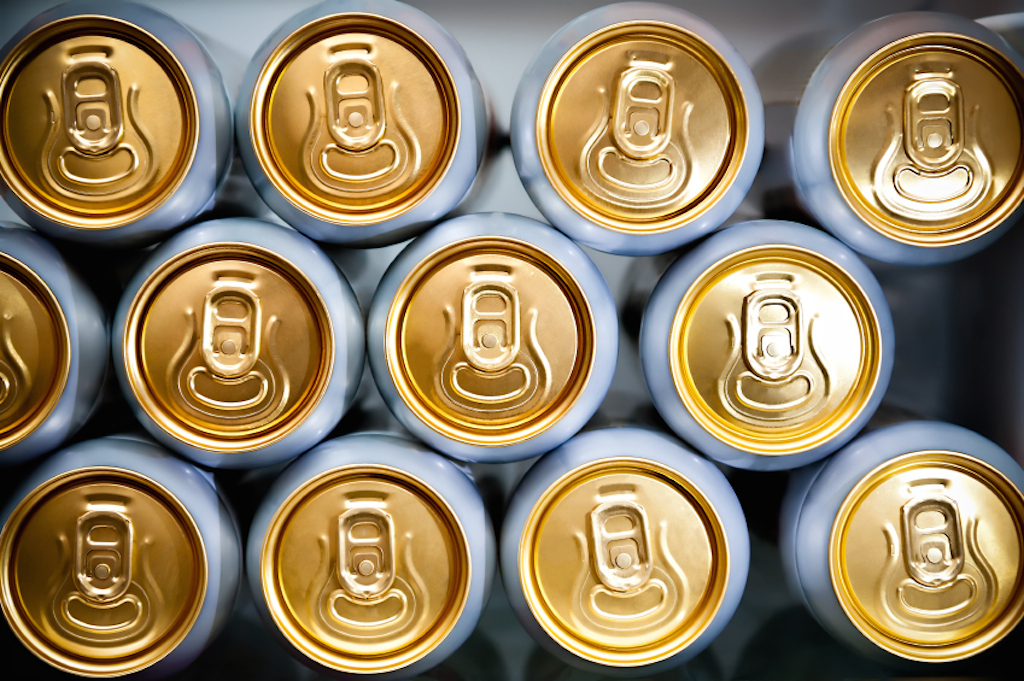
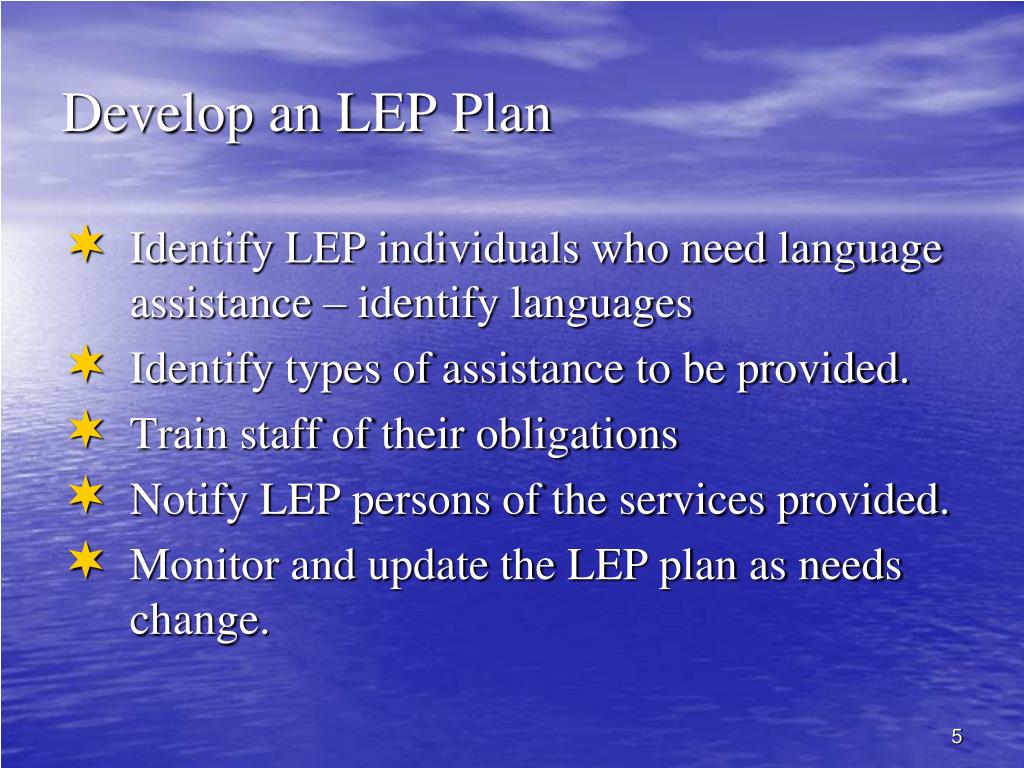
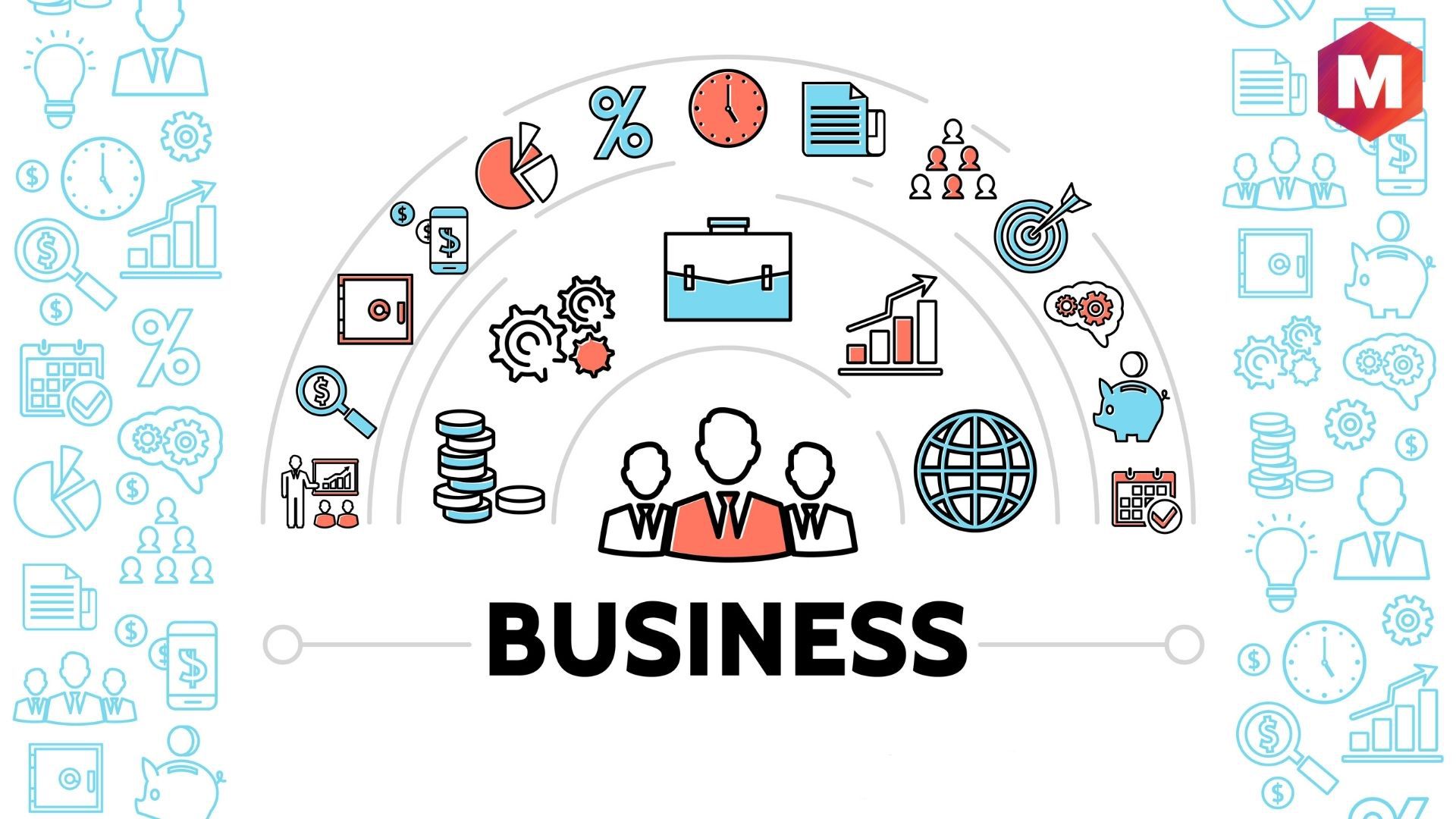
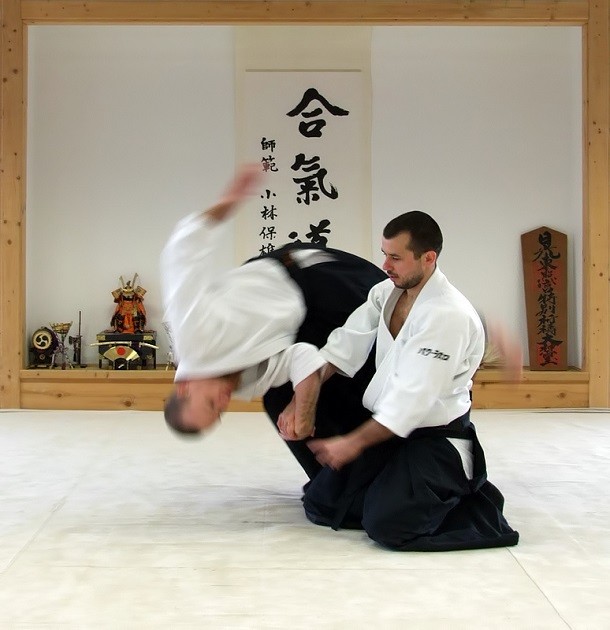
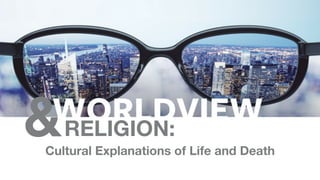
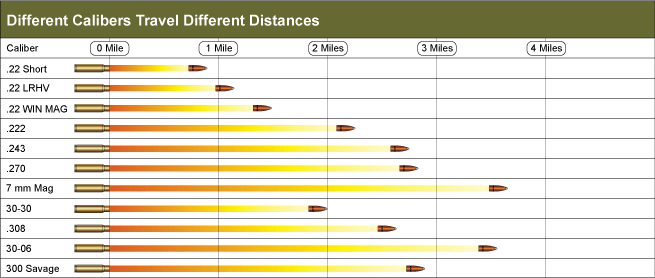